Magnetosphere di Giove – Wikipedia
The Magnetosphere di Giove It is the largest and most powerful of all the magnetospheres of the planets of the Solar System, as well as the largest structure of the Solar System itself not belonging to the Sun: it extends in fact in the external sun system for many times the radius of Jupiter and reaches a maximum size which can exceed the orbit of Saturn. [first] If he were visible to the naked eye from the earth, he would have an apparent extension superior to the diameter of the full moon, [2] despite its great distance.

The magnetic field of Jupiter preserves its atmosphere from the interactions with the sun wind, a plasma flow emitted by our star, deflecting it and creating a distinct region, called magnetosphere, consisting of a plasma of composition very different from that of the sun wind. [3] Although it has a more flat shape than the Earth’s magnetosphere, the Gioviana magnetosphere has an intensity of a higher order of magnitude; The field that feeds is generated by whirlwind motions inside the metal hydrogen layer which constitutes the internal cloak of the planet. [4] The Galileian satellite, known for its intense volcanic activity, contributes to fueling the magnetosphere Gioviana generating an important plasma bull, [5] which loads and strengthens the magnetic field forming the structure called Magnetodic ; [6] It follows that the Gioviana magnetosphere, in spite of the terrestrial one, is fueled by the planet itself and by a satellite rather than the sun wind. The strong currents circulating in the magnetosphere generate intense radiation bands similar to the Van Allen Terrestrial bands, but thousands of more powerful times; [7] These forces generate perennial auror around the planet’s poles and intense variable radio emissions that effectively make Jupiter a weak radio pulsar. [8]
The interaction of the energy particles with the surface of the Galilean Lunes major considerably affects the chemical and physical properties of the magnetosphere, also influenced by the subtle system of rings that orbit around the planet. [9]
The existence of the magnetosphere of Jupiter was hypothesized starting from the radio observations conducted in the 1950s and was studied for the first time in detail by the Pioneer 10 probe in 1973; Since then it has been analyzed seven times by as many probes. [3]
The first evidence of the existence of a magnetic field around Jupiter was in 1955 when the radio decametric emission (DAM) was discovered; [ten] Since the spectrum of the Dam extends up to 40 MHz, the astronomers concluded that the planet possessed a magnetic field with a force of about 0.001 Tesla (T), corresponding to 10 Gauss (G). [11] Later, in 1959, the observations of the part of the radio spectrum to the microwave led to the discovery of the decimetric radiation Gioviana (Dim), which is emitted by the relativistic electrons blocked in the radiation belts; [twelfth] These synchronous emissions were used to estimate the number and energy of the electron population around Jupiter and allowed an increase in the values of the strength of the magnetic field. [5] The modulation of the Dam Gioviane emissions by the satellite I (called I-Dam) was discovered in 1964; His observations allowed to precisely determine the rotational period of Jupiter. [13] The definitive discovery of the magnetic field Gioviano took place in 1973, when the Pioneer 10 spatial probe flew near the planet. [14]
Main features in comparison with the geomagnetic field [ change | Modifica Wikitesto ]
Parameter | Jupiter | Earth |
---|---|---|
Ray of the planet (R p , in km) | 71.398 | 6.371 |
Rotation period (in hours) | 9.9 | 24 |
Field intensity to the equator (in microtesla – μT -) | 428 | thirty first |
Moment of the dipole (in terrestrial units) | 18,000 | first |
Inclination of the magnetic dipole (in °) | ten | 11.3 |
Distance from magnetopause (R p ) | 50–100 | 8–10 |
Potenza in Input (In Treatment – TW -) | 100 | about 1 |
Solar wind density (in cm −3 ) | 0.4 | ten |
Intensity of the Solar Magnetic Field (in Nanotesla – NT -) | first | 6 |
Dominant ionic species | H + , O n+ , S n+ | H + , O + |
Unlike the earth’s magnetic field, which is generated by currents, similar to a dynamo, iron and nickel fused in the external nucleus, the magnetic field of Jupiter is produced inside the metal hydrogen layer surrounding its nucleus. [4] Like the terrestrial one, the Gioviano magnetic field is a dipole, with a north and a magnetic south placed at the ends of a single magnetic axis; [16] However, unlike what happens for our planet, the magnetic North of Jupiter is located in the northern hemisphere and the south pole in the southern hemisphere. [N 1] [17] The Gioviana magnetosphere also presents a development in multipoli (quadrupolo, ottupolo etc.), which decrease in intensity of an order of magnitude from a level to the higher one. [16]
While the geomagnetic field has a “drop” shape, the Gioviana magnetosphere is more crushed, more similar to a disc, and periodically oscillates on its axis. [18] The dipole axis is inclined by 10 ° compared to the rotation axis of the planet, as well as the magnetic axis of our planet is inclined by 11.3 ° compared to the rotation axis. [14] [16] The intensity of the field to Jupiter’s equator is about 420 μT (4.2 g), which corresponds to a moment of the dipole of about 1.5 × 10 20 T·m 3 ; The magnetic field of Jupiter is therefore 10 times more intense than the terrestrial one, and its moment of the magnetic dipole 18,000 times higher. [4] The magnetic field of Jupiter revolves at the same speed of the planet’s cloak, in 9 h 55 m, and is rather stable: in fact, consistent changes in intensity or structure have not been observed from the first measurements obtained thanks to the probes of the Pioneer program in the mid -years seventy. [16]
Shape and size [ change | Modifica Wikitesto ]

The magnetic field of Jupiter preserves its atmosphere from the interactions with the sun wind, a plasma flow emitted by our star, deflinging it and creating a distinct region, called magnetosphere, consisting of a plasma of composition very different from that of the sun wind; [3] The gap present between the plasma of the sun wind and the magnetospheric plasma takes the name of magnetopause and is located at a distance from the planet between 45 and 100 times its radius (the radius of Jupiter – R J – Vale 71,492 km) depending on the period of the solar cycle. [3] [19] Beyond magnetopause (at an average distance of 84 R J from the planet) is the bow shock, the point where the wind flow is deflected by the magnetic field; [20] [21] The region between bow shocks and magnetopause takes the name of magnetosheath. [3] The extension of the planet magnetosphere is such that, if it were visible to the naked eye from our planet, it would appear much larger than the full moon. [2]
On the opposite side, the sun wind draws the magnetic field of the planet in a long coda magnetic, whose extension can arrive far beyond the orbit of Saturn. [first] Its structure is very similar to the terrestrial one; It is made up of two lobes, whose magnetic field focuses in opposite directions: the field of the northern lobe focuses away from the planet, while the southern lobe points towards it. The lobes are divided by a weak layer of plasma called widespread current. [first] The magnetic tail acts as a channel for the plasma particles of the solar wind that manage to penetrate the internal regions of the magnetosphere, which heat up forming radiation bands at a distance of less than 10 r J from the top of the clouds. [7]
The magnetosphere of Jupiter is conventionally divided into three parts: the internal magnetosphere, intermediate and external. The internal magnetosphere is located at a distance of less than 10 r J from the planet; The magnetic field inside remains substantially dipolar, since every contribution from the currents that flow from the equatorial magnetosphere plasma is small. In the intermediate regions (between 10 and 40 r J ) and external (over 40 R J ) The magnetic field is no longer dipola and is seriously disturbed by its interactions with the sun -plasma. [3]
The role of me in food the Gioviana magnetosphere [ change | Modifica Wikitesto ]
Although overall the shape of the magnetosphere of Jupiter resembles the terrestrial one, near the planet its structure is very different. [19] The moon, characterized by an intense volcanic activity, is a powerful source of plasma that fills the magnetosphere of the mother planet of about 1,000 kg of new material every second. [5] The strong volcanic eruptions on the surface of the satellite emit a large amount of sulfur dioxide (I know 2 ), of which a small part dissociate itself in the constituent atoms which, ionizing itself due to the ultraviolet solar radiation, produce the cations S + , O + , S ++ and the ++ . [22] These ions manage to abandon the atmosphere of the satellite, going to constitute the planes, a plasma bull, which reaches a temperature of 100,000-1,000 king, far lower than the one reached in the radiation bands (100 million Kelvin). [5] The plasma in the bull is forced to a co-rotation with Jupiter, and therefore both share the same period of rotation. [23] The bull of I therefore changes the dynamics of the Gioviana magnetosphere in a conspicuous way. [24]

The electrical conductivity of the plasma inside the bull is not infinite; Consequently, the plasma slowly tends to move away from the planet. The main escape mechanisms are the diffusion and instability between the offices. [23] While the plasma moves away from the planet, the radial currents that flow inside them increase their speed (keeping co-rotation), which involves an increase in the kinetic energy of the plasma due to the energy of the rotation of the planet. [3] The density of the plasma is significantly variable inside the magnetosphere: the number of plasma particles ranges from a maximum of 2,000 per cm³ in the bull of I up to about 0.2 per cm³ at a distance of 35 R J ; [25] In this sense, the Gioviana magnetosphere is enhanced by the rotation of the planet, while the Earth’s magnetosphere is mainly reinforced by the solar wind. [24] However, in the intermediate magnetosphere (at distances greater than 10 r J from the planet) the co-rotation gradually is stupid and the plasma begins to rotate more slowly than the planet; [3] at distances greater than 40 R J , in the external magnetosphere, this plasma flees from the magnetic field and leaves the magnetosphere along the magnetic tail, [26] probably in the form of an unpublished planetary wind . [22] The coldest and densest plasma in motion to the outside is replaced by a less dense and warmer plasma (200 million k or higher) coming from the external magnetosphere, [25] Which, as Jupiter approaches Jupiter, undergoes adiabatic heating giving rise to the radiation bands of the internal magnetosphere, which constitute the main source of radio emission of the planet. [5]
The centrifugal force of co-coating plasma draws up the field lines forming, at a distance greater than 20 r J from the planet, a flattened structure known as magnetic disc or Magnetodic . [6] This magnetisk has a weak widespread current in correspondence with the magnetic equator; [22] The lines of the field point in the opposite direction to the planet above this floor and towards it below the floor. [19] The Gioviana magnetosphere, strongly fueled by the plasma of I, expands enormously in width, since the magnetisk creates an additional pressure that balances the pressure of the sun wind. [20] If I was not exactly in that position in the Jupiter system, the distance between the top of the clouds of the planet and the magnetopause would be enormously less: 42 r J against the royal 75 r J medium. [3] So, as abundantly seen, the magnetosphere of the gaseous giant is dominated by the heavy plasma of I and is enhanced by the rotation of the planet, while the sun wind constitutes only a secondary source of plasma and energy, [24] even if it supplies the high energy protons system. [5]
Forces and currents [ change | Modifica Wikitesto ]

As already seen, the main activator of the Gioviana magnetosphere is the rotation of the planet; [27] When it rotates, its ionosphere moves relatively to the dipolar magnetic field of the planet. Since the moment of magnetic dipole focuses in the same direction as rotation, [17] Lorentz’s strength, which appears as a result of this bike, transports electrons, negatively loaded, towards the poles, while the cations are headed towards the equator; [28] Consequently, the poles accumulate negative charges while the regions close to the equator become positive. Although the magnetosphere of Jupiter is full of highly conductive plasma, the electrical circuit thus constituted closed closed; [28] Electric currents follow the trend of the lines of the magnetic field: they flow from the lower latitudes of the ionosphere towards the widespread plasma (Birkeland currents), then they move away from the planet through the plasma and then, finally, return to the planetary ionosphere after crossing the external magnetosphere. The radial current interacts with the planetary magnetic field and the power of Lorentz resulting accelerates the magnetic plasma in the direction of the rotation. This is the main mechanism that maintains magnetospheric plasma in co-rotation. [28]
The current that comes from the ionosphere, called direct current , is more intense if the corresponding part of widespread plasma rotates more slowly than the planet. [28] As mentioned before, the co-rotation stops in the region between 20 and 40 r J from Jupiter; This region corresponds to magnetisk, in which the field lines appear very developed in width. [29] The current that pours into the magnetisk originates in an area of the ionosphere between 15 ° and 17 ° by the magnetic poles; The almost circular area thus described corresponds to the main auroral regions [30] (look down). The return current coming from the most external regions of the magnetosphere (over 50 R J ), penetrates the ionosphere to the poles, closing the electrical circuit; The total radial current of the planet magnetosphere has an estimated intensity on 60-140 million amp (a). [28] [thirty first]
Another important current present in the magnetosphere of Jupiter, which reaches intensity of 160 million a, [3] It is the azimutal ring current, [32] which flows through the equatorial plasma in the same direction as the rotation of the planet. The strength of Lorentz which results from the interaction of this current with the magnetic field prevents co-crop plasma from chairing away from the planet. [3] [thirty first]
In the Gioviana magnetosphere there are other minor currents: the widespread neutral current, which passes inside the widespread plasma in the same direction as the rotation of the planet; the tail currents, proper to the lobes of the magnetic tail, which move in the opposite direction to the rotation; The currents of the magnetopause (also called Chapman-Ferraro currents), which flow along the side exposed to the sun in the opposite direction to the rotation. All these currents contribute to preserving the configuration of the Gioviana magnetosphere by interacting substantially with the sun wind. [17]
Plasma transfer [ change | Modifica Wikitesto ]

The main problem encountered in deciphering the dynamics of the Gioviana magnetosphere concerns the transfer of the heavy cold plasma from the bull of I (at 6 r J from the planet) up to distances greater than 100 r J , in Piena Magnetosphere Song. [29] The exact mechanism is not yet known, but it is assumed that it is a result of the spread of plasma for intercaric instability. The process taken into consideration is very similar to the instability of Rayleigh-Taylor in hydrodynamics: [23] In the case of the Gioviana magnetosphere, the centrifugal force plays the same role played in the instability of the force of gravity; The heavy liquid is the cold and dense plasma of the Toroid, while the light liquid is the hottest and least dense plasma of the external magnetosphere. [23] The instability causes an exchange between the plasma flows full of plasma of the internal regions and those of the external regions of the magnetosphere: the “lively” empty flow pipes move towards the planet, removing the heavy pipes full of the plasma of I and to confine them in the external areas. [23] The intercaric exchange of flow pipes is a form of magnetospheric turbulence. [33]
This hypothetical model was partially confirmed by the data of the Galileo probe, which identified the regions in which the density of the plasma was markedly reduced and others, inside the magnetosphere, in which the intensity of the field was higher than in the rest of the magnetosphere ; [23] These low density regions could correspond to the empty flow pipes arriving from the external magnetosphere. In the intermediate magnetosphere, the probe has identified so -called Injection events , which take place whenever the hot plasma of the external magnetosphere suddenly penetrates the magnetisk, causing an intense flow of energy particles and locally reinforcing the magnetic field. [34]
The cold plasma transport mechanisms to external regions are not yet well known; However, it is assumed that when the flow of flow of the cold plasma of Io reach the external magnetosphere, they meet a reconnection process, which separates the magnetic field from the plasma. [29] These then return to the internal magnetosphere stuffed with the warm and not very dense plasma of the external regions, while the cold plasma is probably ejourished along the magnetic tail in the form of plasmoids (vast plasma bubbles). The reconnection processes would correspond to the global reconfiguration events observed by Galileo, which take place regularly every 2–3 days. [35] These events usually include rapid and chaotic variations of the intensity and the direction of the magnetic field, together with sudden changes in the motorcycle of the plasma, which with a certain frequency ceases to co-strot and begins to flow outwards. These phenomena were observed mainly on the night part of the magnetosphere, in correspondence with the albeggiant regions. [35]
The reconnection events are analogous to the magnetic subtemptste of the Earth’s magnetosphere, [29] But they differentiate them for the causes. The terrestrial subtemptste are caused by the release, through an event of reconnection in the neutral plasma, of the energy of the solar wind stored in the magnetic tail, accompanied by the creation of a plasmoid that moves along the tail. [36] On the contrary, in the magnetosphere of Jupiter, these storms originate when the rotational energy, stored in the magnetisk, is released through the formation of a plasmoid that separates from the disc. [35]
Polar Aurore [ change | Modifica Wikitesto ]

Jupiter shows brilliant and persistent aurors on both poles. Unlike the terrestrial bumps, which are temporary and which are manifested above all in the periods of maximum solar activity, Jupiter’s auror are permanent, although their intensity is not constant, but various day by day. There are three main characteristics: the main oval, narrow (less than 1000 km) but brilliant circular areas placed about 16 ° by the magnetic poles; [37] the auroral spots of the satellites, which correspond to the “footprints” left by the lines of the magnetic field that connect their ionosfers with the ionosphere of the mother planet; The transient polar emissions, located within the main ovals. [37] [38] Although they have been analyzed in almost all the wavelengths (λ) of the electromagnetic spectrum, including X -rays (up to 3 Kev), the auroral emissions appear far more bright in the average infrared (at λ 3–4 µm e 7–14 µm) and in the distant ultraviolet (λ 80–180 nm). [39]
The main oval are the predominant formation in the Aurore Gioviane; they have a very stable form and location, [38] But their intensity is strongly modulated by the pressure exerted by the solar wind: in fact, the more intense the sun wind, the weaker the ear. [40] As already mentioned before, the main ovals are fueled by the strong influx of electrons accelerated by the electric potential that is established between the plasma of the magnetisk and the Gioviana Ionosphere; [41] This current maintains the plasma of the magnetisk magnetisk in co-rotation with the planet. [29] Electrons have energies of the order of the 10–100 Kev and penetrate deeply in the Gioviana atmosphere, ionizing and exciting molecular hydrogen and giving rise to an intense ultraviolet emission. [42] L’ERGIA TOTOE ACCUMULATA DALLA IONOSFERA AMMONTA A 10-100 TREATMENT (TW); [43] In addition, the currents that penetrate the ionosphere heat it by joule effect, which frees a quantity of energy, equal to 300 Two Two, responsible for the strong infrared emission of the aurore and, in part, of the heating of the planetary thermosphere. [44]
Emission | Jupiter | Macchia of me |
---|---|---|
Radio (come, <0.3 MHz) | ~1 GW | ? |
Radio (HOM, 0,3–3 MHz) | ~10 GW | ? |
Radio (DAM, 3–40 MHz) | ~100 GW | 0,1–1 GW (Io-DAM) |
And (idrocarburi, 7-14 μm) | ~40 TW | 30–100 GW |
And (h 3 + , 3–4 μm) | 4–8 TW | |
Visible (0.385-1 μm) | 10–100 GW | 0,3 GW |
UV (80–180 nm) | 2–10 TW | ~50 GW |
X -rays (0.1–3 Kev) | 1–4 GW | ? |
The spots are the result of the interactions of the planetary magnetosphere with that of three of the four Medici or Galileian satellites: I, Europe and Ganimedes; The fourth Galilean moon, Callisto, also think that, gives rise to a stain, but this would be unnoticed as coinciding with the main auroral oval. [forty six] These spots originate because the co-rotation of the plasma is slowed down near the planet’s satellites. The brightest stain belongs to I and it is believed that it is related to a flow of waves of Alfvén directed by the Ionosphere Gioviana towards that of the satellite. The stains of Europe and Ganimede appear much weaker since the two moons, in spite of me, are only weak sources of new magnetospheric plasma, which originates for the sublimation of the ice present on their surface. [47]
Sporadically, inside the main ovals, brilliant arches and spots appear; These transient phenomena are believed to be related to interactions with the sun wind. [38] The field lines in this region are willing to form the polar cuspids of the magnetosphere and magnetic tail. Auroral polar emissions are similar to those observed around the terrestrial poles: both manifest themselves when the electrons are accelerated towards the atmosphere of the planet with electric potential during the reconneys of the solar magnetic field with the magnetic field of the planet. In the case of the earth, the recirculation of the magnetic flow in the stretch exposed to the sun radiation of the magnetosphere and the magnetic tail takes the name of the dungey cycle; It is believed that a similar cycle also exists in the magnetosphere of Jupiter. [29] The emission spectrum in X -rays by the aurore shows the lines of oxygen and highly ionized sulfur, which probably appear when the highly energetic ions (hundreds of Kev) of O and S precipitate in the polar regions of the planetary atmosphere. The origin of this precipitation is still unknown. [48]
Radio emission and modulation of the issue [ change | Modifica Wikitesto ]
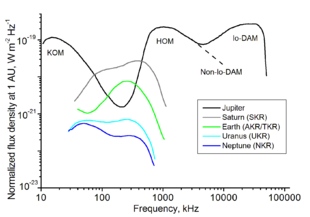
Jupiter is a powerful radio -based radio in the relatively extensive band of the radio spectrum whose frequency ranges from some Kilohertz (KHz) to a few dozen megahertz (MHz). The waves frequency at about 0.3 MHz (λ> 1 km) are called GIOVIANA MILOMETRIC RADIATION O Kom; those with frequencies between 0.3 and 3 MHz (100 <λ <1000 m) are called hectometric radiation or Hom; The frequency waves included in the range 3–40 MHz (10 <λ <100 m) are defined Decametric radiation or Dam. The latter was the first to be observed through land equipment and its periodicity of about ten hours (similar to the rotation of the planet) made it possible to understand that it was originating from the giant planet. The most intense portion of the decametric emission, which is linked to I and the IO-Joves plasma currents system, takes the name of Io-Dam; [49] The non-deam is decidedly weaker than the I-DAM, and therefore represents the high frequency tail of the HOM emission. [49]

Most of these emissions are believed to originate through a mechanism said Cyclotron Maser Instability , Maser Instability of Cyclotron, which originates near the auroral regions, in which the electrons “bounce” back and forth between the poles. The electrons, which are involved in the generation of the radio signal, are probably attributed to transporting the current from the poles of the planet to magnetisk. [50] Usually the intensity of Journey radio emissions varies weakly over time; However, the planet periodically emits short but intense flashes of radiation (called flashes S), which come to overlook all the other components of the radio emission. The overall power emitted by the DAM component amounts to about 100 GW, while the sum of the powers of the Hom/Kom emission does not much exceeds 10 GW; For comparison, the total radio emission of our planet is about 0.1 GW. [49]
A particularly interesting feature of the Gioviana magnetosphere consists of a strong periodic modulation of the radio and particle emission which corresponds to the period of rotation of the planet, a behavior that has affinity with that of the pulsar. [8] This modulation is probably related to Asymmetries in the magnetosphere of the planet, presumably caused by the inclination of the magnetic moment compared to the rotation axis, as well as by magnetic anomalies related to the high latitudes. The physics that governs the radio emission of Jupiter is similar to that of the radio button, and the difference depends only on the energy stairs; As a result, Jupiter can be considered as a small radio radio. [8] However, the fact that the radio emission of the planet is strongly depends on the pressure of the solar wind is taken into consideration and, therefore, on the solar activity itself. [49]
In addition to the great wavelength radiation, Jupiter also emits cyclotron radiation (known as decimetric radiation ), with frequencies of the order of 0.1–15 GHz, [11] which is the braking radiation of the relativistic electrons (with energy of the order of 0.1-100 MEV) bound in the internal radiation bands of the planetary magnetosphere. This radiation is very well known and studied and has been used since the early sixties to study the structure of the magnetic field and the radiation bands of the planetary magnetosphere. [51]
Jupiter’s magnetosphere expels high energy electrons and ions flows (of the order of some tens of mev), which travel in the space also reaching the terrestrial orbit; [52] These flows are highly colored and vary, such as radio emission, according to the rotation period of the planet. [8]
Interactions with rings and natural satellites [ change | Modifica Wikitesto ]
The vast Gioviana magnetosphere incorporates its system of rings and the orbit of all four Galileian satellites. [9] By orbit by near the magnetic equator, these bodies act as a source and dispersers of magnetospheric plasma, while the energy particles from the atmosphere interact with their surface, exposing material and altering their properties. [53] The co-rotation of the plasma with the planet is an indication of the fact that it preferably interacts with the hemisphere trailing (i.e. that opposite to the hemisphere which is oriented according to the verse of the orbital motion, called the hemisphere leading ) of the moons, causing a remarkable hemispherical asymmetry; [54] In addition, the great magnetic fields of the moons affect and locally alter the magnetic field John. [9]


The interactions between the magnetic field of Jupiter and the Satellites Callisto (above) and Ganimede (below); The latter presents a discreet magnetic field of an endogenous nature.
Near Jupiter, planetary rings and small moons absorb high energy particles (higher than 10 Kev) with radiative bands; [55] This creates voids in the spatial and angular distribution of the bands themselves and influences the radiation of synchrotone decametric. In fact, the existence of the rings of Jupiter was hypothesized for the first time on the data collected by the Pioneer 11 probe, which found a substantial quantity of high energy ions in a stilliform space (“drip”) near the planet. [55] The planetary magnetic field strongly influences the motion of the sub-Micrometric particulate of the rings, which acquires an electric charge under the influence of the ultraviolet radiation of the sun; Their behavior is similar to that of co-cring ions. [56] It is believed that the resonant interaction between co-circulation and orbital motion is responsible for creating the ring of Alone, the innermost of the planet’s rings system (located between 1.4–1.71 r J ), which consists of sub-Micrometric particulates arranged on strongly inclined and eccentric orbits. [57] The matter of this ring is originally from the main ring: when in fact these grains slide more internally, their orbit is modified by the strong resonance Lorentz 3: 2, noticeable at 1.71 r J , which increases their inclination and eccentricity; Another resonance Lorentz 1: 2 to 1.4 r J defines the internal edge of the Alone ring. [58]
All Galilean moons show soft atmospheres with superficial pressures between 0.01 and 1 nanobar, which manage to tolerate ionosfers with electronic density between 1000 and 10,000 particles per cm³. [9] The co-crotal flow of the cold magnetospheric plasma is partially deviated in correspondence with the satellites due to the induced currents within the ionosfers, which create cuneiform structures known as Ali di Alfvén . [59] The interaction of the largest moons with the co-crotal flow is similar to the interaction between the solar wind and planets without magnetosphere such as Venus, although the speed of the particles in the co-crotal flow is often lower than the speed of the sound (speeds They vary between 74 and 328 km/s), which avoids the onset of a bow shock. [60] The pressure of the co-rolling plasma continuously tears of the gas from the atmospheres of the satellites (especially from that of I), some of which are therefore ionized and induced in co-rotation. This process generates bulls, of which the most prominent is the already treated bull of me, plasma and gas near the orbite of the satellites. [9] The Galilean moons therefore constitute the main source of the plasma of the internal magnetosphere and intermediate; However, the energy particles largely are not bound to the Alfvén wings and therefore have free access to the surface of the satellites (except in the case of Ganimedes). [sixty one]
All Galilean moons covered with ice, namely Europe, Ganimede and Callisto, generate magnetic moments induced in response to changes in the magnetic field of the mother planet; These variations in moments create diplars magnetic fields around them, which act to compensate the variations in the field of the surrounding environment. [9] The induction is believed to take place in the subsuperficial layers of salted water, which, thanks to the discoveries of the Galileo probe, are believed to exist below the surface of all the larger frozen moons. [62]
In particular, the interaction of the Gioviana magnetosphere with Ganimede, which presents a discreet intrinsic magnetic field, substantially differs from that with the moons not equipped with a magnetic field. [62] The internal magnetic field of Ganimede “digs” a cavity inside the Gioviana magnetosphere, with a diameter equal to about twice the diameter of the satellite, creating a mini-maker inside the magnetosphere of the planet. The field of the satellite deflects the co-rolling plasma flow, also protecting its equatorial regions, in which the field lines, from energy particles close; However, these can collide with the polar regions, in which the lines are open. [63] A part of these particles is trapped near the satellite equator, creating “miniature” radiation bands; [sixty four] The high energy electrons that penetrate Ganimede’s tenuous atmosphere are also responsible for the weak auror identified in correspondence with the polar regions of the satellite. [63]
The charged particles have a considerable influence on the superficial properties of Ganimede. The plasma emitted by I contains sulfur and sodium ions, [65] who tend to preferentially precipitate on hemispheres trailing of Europe and Ganimede, [66] while on Callisto the sulfur is concentrated above all in the hemisphere trailing [ leading ? ] . [sixty seven] The influence of the plasma could be one of the causes of the other differences that occur between the hemisphere trailing and the hemisphere leading of Medici satellites: in fact, their hemispheres trailing (except that of Callisto) they appear darker than those leading , which could indicate that the former have undergone conditioning by the plasma of the planet magnetosphere. [54] The flow of electrons and energy ions is more isotropic, the layer of ice penetrates by splashing the atoms and molecules from it and causing the radiolisi of water and other compounds: the energy particles free the hydrogen from the water molecules e Oxygen: the latter constitutes a thin atmosphere above the frozen moons, the hydrogen instead escapes the gravitational attraction of the moons by dispersing in space; Among the molecules produced for Radiolisi there are ozone (or 3 ) and hydrogen peroxide (h 2 O 2 ). [68] If organic compounds or carbonate compounds are present among the original molecules, it is possible that carbon dioxide molecules are created (CO 2 ), metanolo (ch 3 Oh) and carbonic acid (h 2 CO 3 ); In the presence of sulfur there are sulfur dioxide (I know 2 ), hydrogen disolfuro (h 2 S 2 ) and sulfuric acid (h 2 SO 4 ). [68] The oxidizing substances produced for Radiolisi (molecular oxygen and ozone) can be trapped inside the ice and brought to the sub-superficial oceans, thus constituting a source of oxygen for any forms of aeroby life. [65]

As of 2009, the planet was reached by eight space probes, which have all contributed to improving the knowledge we have on the Gioviana magnetosphere. The first spatial mission that flowed Jupiter was that of Pioneer 10, in December 1973; [14] Its twin Pioneer 11 visited the planet the following year traveling on a strongly inclined trajectory and transit to it at a distance of 1.6 r j ; This probe provided the best coverage available so far in the internal magnetosphere of the planet. The level of radiation on Jupiter measured by the Pioneer 10 was ten times more powerful than that provided by the manufacturers of the two pioneers, generating the fear that both probes could be damaged in the planet of the planet. However, with some minor damage, Pioneer 11 also managed to cross the almost unscathed magnetosphere, thanks to the fact that the magnetosphere itself was slightly unstable at the crossing point; Despite this, he lost many images of the satellite, since his photographic polarimeter received a series of altered commands caused by radiation. The subsequent and much more advanced Voyager technology used in the homonymous probes was entirely redefined in order to resist large levels of radiation. [18]
The Voyager 1 and Voyager 2 probes arrived near Jupiter respectively in 1979 and 1980, although they traveled mainly on equatorial orbits; Voyager 1 met the plasma bull of Io for the first time, while Voyager 2 discovered the widespread current of the equatorial plan. The next Gioviana mission was Ulysses , which in 1992 broke the polar magnetosphere of the planet. [3]
The Galileo probe, which orbit around Jupiter from 1995 to 2003, provided an almost complete coverage of the magnetic field near the equatorial plan at a distance of 100 R j ; The regions studied include the magnetic field and its clear and dusty sectors. [3] Although Galileo successfully survived in the severe radiative environment of Jupiter, he still suffered some little damage: his gyroscope in fact showed an increase in errors. Sometimes the electric arches that appeared between the rotating and non -rotating parts of the probe caused the fall of the same in safety mode, which led to the total loss of the data collected during the sixteenth, the eighteenth and thirty -three orbit; The radiation also caused the phase movements of the quartz oscillator of the probe. [69]
In 2000, when the Cassini probe flushed the planet, he performed coordinated measurements with Galileo. [3] The last probe to visit Jupiter was the New Horizons in 2007, which led a special investigation by the Gioviana magnetosphere crossing them for a distance of 2500 R j in the direction of its length. [70]
The coverage of the Gioviana magnetosphere, however, remains much more scarn than that of our planet; The knowledge of the processes of the Gioviana magnetosphere will be further improved by the analysis of the data collected by the Mission Juno della Nasa, who entered the orbit around Jupiter on July 5, 2016 and specifically developed to study the magnetosphere of the planet. [3]
- Notes to the text
- ^ However, we must not confuse north and south magnetic, a purely physical concept, with the north pole and the south pole of the planet, a concept of a geographical nature.
- Sources
- ^ a b c Khurana, pp. 17-18 , 2004.
- ^ a b Russell, p. 715 , 1993.
- ^ a b c d It is f g h i j k l m n O p Khurana, pp. 1-3 , 2004.
- ^ a b c d Russel, p. 694 , 1993.
- ^ a b c d It is f Khurana, pp. 5-7 , 2004.
- ^ a b Russell, pp. 1021-1024 , 2001.
- ^ a b Khurana, pp. 6-7 , 2004.
- ^ a b c d Hill , 1995.
- ^ a b c d It is f Kivelson, pp. 2-4 , 2004.
- ^ Burke , 1955.
- ^ a b Zarka, 371–375 , 2005.
- ^ Drake , 1959.
- ^ Zarka, 375–377 , 2005.
- ^ a b c Smith , 1974.
- ^ Blanc, p. 238 (tab. II) , 2005.
- ^ a b c d Khurana, pp. 3-5 , 2004.
- ^ a b c Kivelson, pp. 303-313 , 2005.
- ^ a b Wolverton, pp. 100-157 , 2004.
- ^ a b c Russell, pp. 1015-1016 , 2001.
- ^ a b Krupp, pp. 15-16 , 2004.
- ^ Russell, pp. 725-727 , 1993.
- ^ a b c Krupp, pp. 3-4 , 2004.
- ^ a b c d It is f Krupp, pp. 4-7 , 2004.
- ^ a b c Krupp, pp. 1-3 , 2004.
- ^ a b Khurana, pp. 10-12 , 2004.
- ^ Russell, pp. 1024-1025 , 2001.
- ^ Blanc, pp. 250-253 , 2005.
- ^ a b c d It is Cowley, pp. 1069-76 , 2001.
- ^ a b c d It is f Blanc, pp. 254-261 , 2005.
- ^ Cowley, pp. 1083-87 , 2001.
- ^ a b Khurana,, pp. 13–16 , 2004.
- ^ Kivelson, pp. 315-316 , 2005.
- ^ Russell , 2008.
- ^ Krupp, pp. 7-9 , 2004.
- ^ a b c Krupp, pp. 11-14 , 2004.
- ^ Russell, p. 1011 , 2001,
- ^ a b Palier, pp. 1171-73 , 2001.
- ^ a b c Bhardwaj, pp. 311-316 , 2000.
- ^ Bhardwaj, p. 342 , 2000.
- ^ Cowley, pp. 49-53 , 2003.
- ^ Bhardwaj, pp. 316-319 , 2000.
- ^ Bhardwaj, pp. 306-311 , 2000.
- ^ Bhardwaj, p. 296 , 2000.
- ^ Miller, pp. 335-339 , 2005.
- ^ Bhardwaj, tavole 2 e 5 , 2000.
- ^ Clarke , 2002.
- ^ Blanc, pp. 277-283 , 2005.
- ^ Elsner, pp. 419-420 , 2005.
- ^ a b c d Zarka, pp. 20,160–168 , 1998.
- ^ Zarka, pp. 20,173–181 , 1998.
- ^ Zarka, pp. 384-385 , 2005.
- ^ Krupp, pp. 17-18 , 2004.
- ^ Johnson, pp. 1-2 , 2004.
- ^ a b Johnson, pp. 3-5 , 2004.
- ^ a b Burns, pp. 1-2 , 2004.
- ^ Burns, pp. 12-14 , 2004.
- ^ Burns, pp. 10-11 , 2004.
- ^ Burns, pp. 17-19 , 2004.
- ^ Kivelson, pp. 8-10 , 2004.
- ^ Kivelson, pp. 1-2 , 2004.
- ^ Cooper, pp. 137,139 , 2001.
- ^ a b Kivelson, pp. 10-11 , 2004.
- ^ a b Kivelson, pp. 16-18 , 2004.
- ^ Williams, p. 1 , 1998.
- ^ a b Cooper, pp. 154-156 , 2001.
- ^ Johnson, pp. 15-19 , 2004.
- ^ Hibbitts, p. 1 , 2000.
- ^ a b Johnson, pp. 8-13 , 2004.
- ^ Fieseler , 2002.
- ^ KROUPH, P. 216 , 2007.
General titles [ change | Modifica Wikitesto ]
- ( IN ) Albrecht Unsöld, The New Cosmos , New York, Springer-Verlag, 1969.
- H. L. Shipman, The restless universe. Guide to observation with the naked eye and with the telescope. Introduction to astronomy , Bologna, Zanichelli, 1984, Isbn 88-08-070-5.
- ( IN ) Stephen Hawking, A Brief History of Time , Bantam Books, 1988, ISBN 0-553-17521-1.
- H. Reeves, Cosmic evolution , Milano, Rizzoli–Bur, 2000, ISBN 88-17-25907-1
- AA.VV, The Universe – Great encyclopedia of astronomy , Novara, De Agostini, 2002.
- J. Gribbin, Encyclopedia of astronomy and cosmology , Milan, Garzanti, 2005, ISBN 88-11-50517-8.
- W. Owen, et al, Illustrated atlas of the universe , Milan, The traveler, 2006, ISBN 88-365-3679-4.
- M. Rees, Universe. From the Big Bang to the birth of the planets. From the solar system to the most remote galaxies , Milan, Mondadori Electa, 2006, pp. 512.
Specific titles [ change | Modifica Wikitesto ]
On the sun system [ change | Modifica Wikitesto ]
- M. Hack, Discovering the Solar System , Milan, Mondadori Electa, 2003, pp. 264.
- F. Biafore, Traveling in the sun system. A path in space and time in the light of the latest discoveries , Group B, 2008, pp. 146.
- ( IN ) Various, Encyclopedia of the Solar System , Group B, 2006, pp. 412, ISBN 0-12-088589-1.
On the planet [ change | Modifica Wikitesto ]
- ( IN ) Bertrand M. Peek, The Planet Jupiter: The Observer’s Handbook , Londra, Faber and Faber Limited, 1981, ISBN 0-571-18026-4, , OCLC 8318939.
- ( IN ) Eric Burgess, By Jupiter: Odysseys to a Giant , New York, Columbia University Press, 1982, ISBN 0-231-05176-X.
- ( IN ) John H. Rogers, The Giant Planet Jupiter , Cambridge, Cambridge University Press, 1995, ISBN 0-521-41008-8, , OCLC 219591510.
- ( IN ) BeeBe Reta, Jupiter: The Giant Planet , 2ª ed., Washington, Smithsonian Institute Press, 1996, ISBN 1-56098-685-9.
- ( IN ) AA.VV., The New Solar System , a cura di Kelly J. Beatty; Carolyn Collins Peterson; Andrew Chaiki, 4ª ed., Massachusetts, Sky Publishing Corporation, 1999, ISBN 0-933346-86-7, , OCLC 39464951.
- ( IN ) D. C. Jewitt; S. Sheppard ; C. Porco, F. Bagenal; T. Dowling; W. McKinnon, Jupiter: The Planet, Satellites and Magnetosphere ( PDF ), Cambridge, Cambridge University Press, 2004, ISBN 0-521-81808-7 (archived by URL Original on June 14, 2007) .
- ( IN ) Linda T. Elkins-Tanton, Jupiter and Saturn , New York, Chelsea House, 2006, ISBN 0-8160-5196-8
Scientific publications (in English) [ change | Modifica Wikitesto ]
- B. F. Burke, K. L. Franklin, Observations of a variable radio source associated with the planet Jupiter , in Journal of Geophysical Research , vol. 60, n. 2, 1955, pp. 213–217, DOI: 10.1029/JZ060i002p00213 .
- F. D. Drake, S. Hvatum, Non-thermal microwave radiation from Jupiter , in Astronomical Journal , vol. 64, 1959, p. 329, two: 10.1086/108047 .
- E. J. Smith, L. Davis Jr., D. E. Jones, et.Al. , The Planetary Magnetic Field and Magnetosphere of Jupiter: Pioneer 10 , in Journal of Geophysical Research , vol. 79, 1974, pp. 3501–13, two: 10.1029/JA079i025p03501 .
- C. T. Russell, Planetary Magnetospheres ( PDF ), in Reports on Progress in Physiscs , vol. 56, 1993, pp. 687–732, two: 10.1088/0034-4885/56/6/001 .
- T. W. Hill, A. J. Dessler, Space Physics and Astronomy Converge in Exploration of Jupiter’s Magnetosphere , in Earth in Space , vol. 8, 1995, p. 6. URL consulted on April 18, 2009 (archived by URL Original on May 1, 1997) .
- D. J. Williams, B. Mauk, R. W. McEntire, Properties of Ganymede’s magnetosphere as revealed by energetic particle observations , in Journal of Geophysical Research , vol. 103, A8, 1998, pp. 17,523–534, two: 10.1029/98ja01370 .
- P. Zarka, W. S. Kurth, Auroral radio emissions at the outer planets: Observations and theory , in Journal of Geophysical Research , vol. 103, E9, 1998, pp. 20,159–194, DOI: 10.1029/98je01323 .
- Anil Bhardwaj, G. Randal Gladstone, Auroral emissions of the giant planets ( PDF ), in Reviews of Geophysics , vol. 38, n. 3, 2000, pp. 295–353, doi: 10.1029 / 1998RG000046 .
- C. A. Hibbitts, T. B. McCord, T.B. Hansen, Distribution of CO 2 and SO 2 on the surface of Callisto , in Journal of Geophyscal Reserach , vol. 105, E9, 2000, pp. 22,541–557, DOI: 10.1029/1999JE001101 .
- John F. Cooper, Robert E. Johnson, Barry H. Mauk, et.Al. , Energetic Ion and Electron Irradiation of the Icy Galilean Satellites ( PDF ), in Icarus , vol. 139, 2001, pp. 133–159, two: 10.1006/icar.2000.6498 (archived by URL Original February 25, 2009) .
- S. W. H. Cowley, E. J. Bunce, Origin of the main auroral oval in Jupiter’s coupled magnetosphere–ionosphere system , in Planetary and Space Sciences , vol. 49, 2001, pp. 1067–66, two: 10.1016/S0032-0633(00)00167-7 .
- T. M. Edwards, E. J. Bunce, S. W. H. Cowley, A note on the vector potential of Connerney et al.’s model of the equatorial current sheet in Jupiter’s magnetosphere , in Planetary and Space Science , vol. 49, 2001, pp. 1115–1123, two: 10.1016/S0032-0633(00)00164-1 .
- Laurent Lande, More about the structure of the high latitude Jovian aurorae , in Planetary and Space Sciences , vol. 49, 2001, pp. 1159–73, two: 10.1016/S0032-0633(01)00023-X .
- C. T. Russell, The dynamics of planetary magnetospheres , in Planetary and Space Science , vol. 49, 2001, pp. 1005–1030, two: 10.1016/S0032-0633(01)00017-4 .
- D. Santos-Costa, S. A. Bourdarie, Modeling the inner Jovian electron radiation belt including non-equatorial particles , in Planetary and Space Science , vol. 49, 2001, pp. 303–312, two: 10.1016/S0032-0633(00)00151-3 .
- C. T. Russell, Z. J. Yu, M. G. Kivelson, The rotation period of Jupiter ( PDF ), in Geophysics Research Letters , vol. 28, n. 10, 2001, pp. 1911–1912, DOI: 10.1029/2001GL012917 .
- J. T. Clarke, J. Ajello, J. Ballester, et.Al. , Ultraviolet emissions from the magnetic footprints of Io, Ganymede and Europa on Jupiter ( PDF ), in Nature , vol. 415, 2002, pp. 997–1000, two: 10.1038/415997A .
- P. D. FIESELY, The Radiation Effects on Galileo Spacecraft Systems at Jupiter ( PDF ), in Nuclear Science , vol. 49, n. 6, 2002, pp. 2739–58, doi: 10.1086/108047 (archived by URL Original July 19, 2011) .
- G. R. Gladstone, J. H. WAITE, D. GROUDENT, A pulsating auroral X-ray hot spot on Jupiter , in Nature , vol. 415, 2002, pp. 1000–1003, two: 10.1038/4151000 .
- M. G. Kivelson, K. K. Khurana, R. J. Walker, Sheared magnetic field structure in Jupiter’s dusk magnetosphere: Implications for return currents ( PDF ), in Journal of Geophysical Research , vol. 107, A7, 2002, p. 1116, two: 10.1029/2001JA000251 .
- S. W. H. Cowley, E. J. Bunce, Modulation of Jovian middle magnetosphere currents and auroral precipitation by solar wind-induced compressions and expansions of the magnetosphere: initial response and steady state , in Planetary and Space Sciences , vol. 51, 2003, pp. 31–56, two: 10.1016/S0032-0633(02)00130-7 .
- ( IN ) B. F. Burns; P. D. Simonelli; M. R. Showalter; et al , Jupiter’s Ring-Moon System ( PDF ), in F. Bagenal, T. E. Dowling, W. B. McKinnon (a cura di), Jupiter: The Planet, Satellites and Magnetosphere , Cambridge, Cambridge University Press, 2004, ISBN 0-521-81808-7. URL consulted on April 1, 2009 .
- ( IN ) R. E. Johnson; R. V. Carlson; J. F. Cooper; et al , Radiation Effects on the Surfaces of the Galilean Satellites ( PDF ), in F. Bagenal, T. E. Dowling, W. B. McKinnon (a cura di), Jupiter: The Planet, Satellites and Magnetosphere , Cambridge, Cambridge University Press, 2004, ISBN 0-521-81808-7. URL consulted on April 1, 2009 (archived by URL Original April 30, 2016) .
- ( IN ) K. Khurana; M. G. Kivelson; V. M. vasyliunas; et al , The Configuration of Jupiter’s Magnetosphere ( PDF ), in F. Bagenal, T. E. Dowling, W. B. McKinnon (a cura di), Jupiter: The Planet, Satellites and Magnetosphere , Cambridge, Cambridge University Press, 2004, ISBN 0-521-81808-7. URL consulted on April 1, 2009 .
- ( IN ) M. G. KIVELRON; F. Bagenal; W. S. Kurth; et al , Magnetospheric Interactions with Satellites ( PDF ), in F. Bagenal, T. E. Dowling, W. B. McKinnon (a cura di), Jupiter: The Planet, Satellites and Magnetosphere , Cambridge, Cambridge University Press, 2004, ISBN 0-521-81808-7. URL consulted on April 1, 2009 .
- ( IN ) N. Krupp; V. M. vasyliunas; J. Woch; et al , Dynamics of the Jovian Magnetosphere ( PDF ), in F. Bagenal, T. E. Dowling, W. B. McKinnon (a cura di), Jupiter: The Planet, Satellites and Magnetosphere , Cambridge, Cambridge University Press, 2004, ISBN 0-521-81808-7. URL consulted on April 1, 2009 .
- Mark Wolverton, The Depths of Space , Joseph Henry Press, 2004, ISBN 978-0-309-09050-6.
- M. Blanc, R. Kallenbach, M. V. Erkaev, Solar System Magnetospheres , in Space Science Reviews , vol. 116, 2005, pp. 227–298, two: 10.1007/s11214-005-1958-y .
- R. F. Elsner, B. D. Ramsey, J. H. Waite, et al , X-ray probes of magnetospheric interactions with Jupiter’s auroral zones, the Galilean satellites, and the Io plasma torus ( PDF ), in Icarus , vol. 178, 2005, pp. 417–428, two: 10.1016/j.icarus.2005.06.006 . URL consulted on April 18, 2009 (archived by URL Original on June 19, 2019) .
- Margaret Galland Kivelson, The current systems of the Jovian magnetosphere and ionosphere and predictions for Saturn ( PDF ), in Space Science Reviews , vol. 116, Springer, 2005, pp. 299–318.
- M. G. Kivelson, Transport and acceleration of plasma in the magnetospheres of Earth and Jupiter and expectations for Saturn ( PDF ), in Advances in Space Research , vol. 36, 2005, pp. 2077–89, two: 10.1016/J.ASR.2005.05.104 .
- Steve Miller, Alan Aylword, George Milliword, Giant Planet Ionospheres and Thermospheres: the Importance of Ion-Neutral Coupling , in Space Sci.Rev. , vol. 116, 2005, pp. 319–343, two: 10.1007/s11214-005-1960-4 .
- P. Zarka, W. S. Kurth, Radio wave emissions from the outer planets before Cassini , in Space Science Reviews , vol. 116, 2005, pp. 371–397, two: 10.1007/s11214-005-1962-2 .
- J. D. Nichols, S. W. H. Cowley, D. J. McComas, Magnetopause reconnection rate estimates for Jupiter’s magnetosphere based on interplanetary measurements at ~5 AU , in Annales Geophysicae , vol. 24, 2006, pp. 393–406.
- Norrted curve, New Surprises in the Largest Magnetosphere of Our Solar System , in Science , vol. 318, 2007, pp. 216–217, two: 10.1126/science.1150448 .
- C. T. Russell, K. K. Khurana, C. S. Arridge, M. K. Dougherty, The magnetospheres of Jupiter and Saturn and their lessons for the Earth ( PDF ), in Advances in Space Research , vol. 41, 2008, pp. 1310–18, two: 10.1016/J.ASR.2007.07.037 . URL consulted on April 18, 2009 (archived by URL Original February 15, 2012) .
Recent Comments